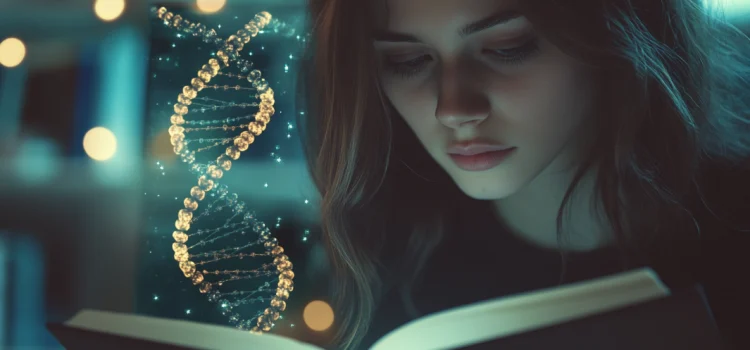
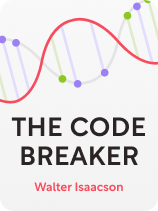
This article is an excerpt from the Shortform book guide to "The Code Breaker" by Walter Isaacson. Shortform has the world's best summaries and analyses of books you should be reading.
Like this article? Sign up for a free trial here.
Have you heard of CRISPR and wondered what all the fuss is about? How could this groundbreaking technology change the world as we know it?
The Code Breaker, a book by Walter Isaacson, explores the fascinating world of CRISPR gene editing technology. The book lays out its development, focusing on Nobel Prize winner Jennifer Doudna’s contributions, and examines the moral quandaries and potential applications of this revolutionary scientific tool.
Continue reading for an overview of this important book.
The Code Breaker Book Overview
The Code Breaker, a book by Walter Isaacson published in 2021, discusses the advent and future of CRISPR, a groundbreaking scientific tool. Isaacson traces its development (focusing on the contributions of scientist and Nobel Prize winner Jennifer Doudna) and explores its significance as a victory for women in science. He also argues that CRISPR has the potential to change life as we know it and explains how, in some ways, it already has.
Isaacson is a journalist who served as the editor of Time magazine and CEO of CNN. He’s also a history professor at Tulane University and author of several popular biographies, including Leonardo Da Vinci, Steve Jobs, and Elon Musk. In 2021, President Joe Biden awarded Isaacson the National Humanities Medal in recognition of his work to bridge the sciences and the humanities.
In our overview, we’ll focus on four of the many threads Isaacson weaves together. In Part 1, we’ll define the biological process known as CRISPR and explain how it works. In Part 2, we’ll discuss the scientists behind CRISPR gene editing technology, with a focus on the life and career of Jennifer Doudna. In Part 3, we’ll explore the moral quandaries that the advent of CRISPR technology presents. Finally, in Part 4, we’ll discuss some of CRISPR’s realized and potential real-world applications.
Part 1: The Biological Process Known as CRISPR
CRISPR is short for “clustered regularly interspaced short palindromic repeats,” which refers to a repetitive pattern inside the DNA of some types of micro-organisms. It’s a natural biological feature that scientists discovered and used to facilitate gene editing. We’ll give some scientific background that will help you understand CRISPR. Then, we’ll explain how scientists discovered CRISPR in nature and figured out how it works.
Scientific Background
To help you understand CRISPR, Isaacson provides some scientific background information on three basic biological units: genes, DNA (deoxyribonucleic acid), and RNA (ribonucleic acid). Let’s explore those now.
Genes
Isaacson traces CRISPR’s development back to the proto-genetic theories of scientists Charles Darwin and Gregor Mendel in the mid-1800s. Darwin, who proposed the theory of evolution, argued that certain traits were passed down through generations via a process called natural selection. He thought reproduction occurred when tiny parts of both parents’ bodies migrated into the egg and sperm and then blended in the offspring, but this wasn’t quite correct. Mendel then showed that some traits are dominant (more likely to appear) and others are recessive (less likely to appear). These findings led other scientists to hypothesize about the existence of genes—molecular units containing inheritable traits.
DNA
Later, scientists discovered that genes are segments of DNA (deoxyribonucleic acid), a molecule inside which inheritable traits are encoded. In the 1950s, scientists Rosalind Franklin, James Watson, and Francis Crick showed that DNA has a double helix structure that can be split in two and replicated—this process is what enables genes to be inherited. Isaacson notes that Franklin’s contributions to the discovery of DNA’s structure were undervalued because of her gender (Watson and Crick were men and have received greater historical recognition).
According to Isaacson, experts long believed that they could begin to solve inheritable medical problems by studying DNA and identifying problematic genes. This led to the formation of the Human Genome Project (an international effort to catalog human DNA) in the 1990s. But Isaacson explains that this approach was lacking—DNA only tells you which genes are present in a cell, not how to alter them.
RNA
Alongside DNA, cells contain a molecule called RNA (ribonucleic acid). Until the 1980s, scientists only knew about one type of RNA: messenger RNA (mRNA), which copies and transmits genetic information. Then, scientists discovered another type of RNA called ribozymes, which act like enzymes (a kind of protein that catalyzes chemical reactions). They also found out that ribozymes could help RNA molecules splice themselves—that is, the molecules could make copies of themselves that automatically cut out certain unnecessary sections. Isaacson explains that this discovery opened the door for scientists like Jennifer Doudna to research how RNA could be used to alter genes.
CRISPR in Nature
Now that you know the fundamental science underlying CRISPR, let’s talk about what CRISPR is and how it works. As a reminder, the label “CRISPR” refers to a repetitive pattern inside DNA. Isaacson explains that scientists in the 1980s noticed that this phenomenon occurred in some bacterial cells, but they weren’t sure what it was or why it mattered. A researcher solved that mystery a few years later—in the bacteria he studied, cells with CRISPR were immune to viral infection, while cells without CRISPR were susceptible to viral infection. Therefore, he dubbed CRISPR a natural antiviral defense mechanism.
Once CRISPR was discovered, scientists set out to decipher how it worked. First, they noticed that the pattern was usually accompanied by enzymes known as Cas enzymes. They theorized that when a virus attacks a bacterial cell, the bacterial cell’s Cas enzymes cut segments of the virus’s genetic material (either DNA or RNA, depending on the virus). Then, the cell copies those segments into the bacteria’s DNA, which results in the DNA pattern known as CRISPR. The next time the virus attacks, the cell “recognizes” the virus because its genes are now encoded in the cell’s DNA—which enables the cell to better defend itself against that specific virus. Isaacson explains that this understanding of how CRISPR and Cas work is correct, but not complete.
Isaacson says in 2012, American biochemist Jennifer Doudna teamed up with French scientist Emmannuelle Charpentier to fully explain how CRISPR-Cas systems defend bacterial cells against invading viruses. They published a paper on how three essential molecules—a Cas enzyme called Cas9 and two kinds of RNA called crRNA (CRISPR RNA) and tracrRNA (trans-activating CRISPR RNA)—work together to facilitate the process. First, tracrRNA creates crRNA, and these molecules combine to produce “guide RNA” (gRNA) and then bind to Cas9. Then, using its matching code as a guide, the gRNA finds the right spot to slice the virus’s DNA. Finally, Cas9 makes a cut in the DNA where the gRNA attached, disabling the virus.
Importantly, in this paper, Doudna and Charpentier also suggested that in the future, scientists could potentially use the CRISPR-Cas9 system to edit human genes.
Part 2: Jennifer Doudna and the Scientists Behind CRISPR Technology
While many scientists played key roles in the development of the gene editing technology known as CRISPR, Isaacson focuses on Jennifer Doudna. We’ll explore how Doudna’s early life and career led her to help develop the CRISPR technology. We’ll also discuss the relative contributions of her colleagues and competitors. Finally, we’ll explain how CRISPR launched Doudna’s career.
Doudna’s Early Life and Career
Doudna was born in 1964 and spent the majority of her childhood in Hawaii. Because she was in the minority of white children there, she felt like an outsider, and she took refuge in Hawaiian nature. Isaacson explains that two adults in Doudna’s life nurtured her fascination with nature: A friend of her parents, who was a biologist, taught her some scientific basics during nature walks, and her father gave her a copy of The Double Helix (the story of how scientists Crick, Watson, and Franklin discovered the structure of DNA). The book excited Doudna for two reasons: First, it inspired her to investigate why the world worked as it does. Second, it taught her that despite what her teachers said, women could be scientists.
Doudna majored in chemistry at Pomona College and went to graduate school at Harvard University. Isaacson explains that at the time, most biologists were preoccupied with studying DNA because they thought it was the next scientific frontier. However, Doudna’s advisor at Harvard encouraged her to focus on RNA. Doudna began exploring ribozyme structure and function, which presaged her work with CRISPR. Then, as a postdoctoral researcher at the University of Colorado, she set out to discover why RNA could be used to edit genes. With the help of her labmate and future husband Jamie Cate, she mapped out RNA’s structure, which helped her understand how it worked.
Isaacson explains that with these discoveries under her belt, Doudna was officially an expert on RNA. The University of California at Berkeley offered her a professorship, and she continued to research RNA in that capacity. There, she focused on RNA interference—a process that she hypothesized could be used for gene editing. Doudna’s research on gene editing took a different turn than she expected, though: When a colleague suggested that she apply her RNA expertise to CRISPR, she tasked her lab with learning how Cas enzymes worked by determining their structure. Once they accomplished that, Doudna moved onto groundbreaking research—she teamed up with Charpentier to clarify how the CRISPR-Cas system works.
Doudna Developed CRISPR Technology Alongside Colleagues and Competitors
Isaacson explains that after Doudna helped discover how CRISPR-Cas9 works in nature, she focused on developing the system into a technology for human use. Doudna played a huge role in the development of CRISPR technology, but she didn’t do it alone—both colleagues and competitors helped her establish this scientific innovation. Let’s discuss the contributions each key player made toward the advent of CRISPR technology.
Doudna Collaborates With Charpentier
Around the same time that Doudna began researching Cas enzymes, other scientists were conducting experiments aimed at preventing viral infections in the bacteria used to make yogurt. They discovered that they could insert CRISPR elements into bacterial cells instead of waiting for cells to naturally develop CRISPR-derived immune defenses. The scientists also confirmed that cells’ CRISPR defenses were inheritable. Based on this discovery, the scientific community concluded that it could potentially use CRISPR to create inheritable alterations to any type of gene.
Doudna and Charpentier turned this potentiality into an actuality, writes Isaacson. They realized that since crRNA determined which genes to cut out of a virus, they could use different versions of crRNA with different targets to edit any segment of DNA they wanted. To simplify this process, they fused together crRNA and tracrRNA to create sgRNA (single-guide RNA) in 2013. The invention of sgRNA transformed CRISPR from a biological process into a gene editing tool. They experimented with single-celled organisms and proved that they could edit genes at will. Isaacson explains that other gene editing tools already existed, but CRISPR gene editing technology quickly displaced them because it was more straightforward and efficient.
Doudna Competes With Zhang (and Other Scientists)
Isaacson explains that although Doudna and Charpentier successfully demonstrated that they could edit the genes of single-celled organisms, it wasn’t immediately clear that the same process could be used in more complex cells (like human cells). As soon as Doudna and Charpentier published their paper on CRISPR gene editing technology, scientists around the world began competing to become the first to use it in human cells. Doudna’s primary competitor in this race was Feng Zhang, a Harvard-educated biochemist with expertise in earlier forms of gene editing technology.
Zhang says that he independently figured out how CRISPR works and used it to edit human and mouse cells by the middle of 2012. But Isaacson explains there are two reasons not to give Zhang credit for the invention of CRISPR gene editing technology: First, according to Zhang’s own records, he didn’t understand how important Cas9 and tracrRNA were to the CRISPR process; Doudna and Charpentier definitely made that discovery. Second, Zhang didn’t create sgRNA (the synthetic molecule that transformed CRISPR from a natural process into a technology)—Doudna and Charpentier did. However, Zhang disagrees that sgRNA is integral to the technology, and Doudna’s lab discovered that sgRNA doesn’t work well for editing human cells.
According to Isaacson, the race to optimize CRISPR gene editing technology for human cells was close. Zhang made modifications to the original version of sgRNA that Doudna and Charpentier developed. His paper was published the same day as another scientist’s paper, and the other scientist’s modifications to sgRNA proved more suitable for human DNA editing than Zhang’s. Later the same month, Doudna published her own paper, which demonstrated another way to apply CRISPR to human cells. Two other scientists published papers on the same topic that year. Since multiple scientists made significant contributions to the application of CRISPR gene editing technology in human cells, it was difficult to say who invented the process.
The War for Patent Rights and Recognition
Isaacson explains that both academia and the biotechnology industry are extremely competitive. In academia, being the first to make a discovery leads to greater prestige and career success. In the biotechnology industry, being first enables you to develop patents.
The competitive nature of scientific research—and the ambiguity surrounding who truly invented the process for human gene editing—led Doudna, Charpentier, and Zhang to become embroiled in a war for patent rights and recognition. Let’s explore how that played out.
In 2013, Doudna, Zhang, and other scientists established a CRISPR-focused medical research company together called Editas Medicine. However, Doudna left the company a few months later due to a conflict: Doudna and Charpentier had applied for a patent together, as had Zhang and his team. Zhang paid to accelerate the application process, so he was granted the patent for CRISPR first. Isaacson says that Doudna felt this was unfair: She believed she and Charpentier were the first to develop the technology, and she thought Zhang’s actions were underhanded and proved him untrustworthy. She left Editas Medicine to join Intellia, an offshoot of a biotechnology company she’d built earlier in her career (Caribou Biosciences).
Meanwhile, conflict was also brewing between Doudna and Charpentier: Charpentier viewed herself as the primary researcher who discovered CRISPR technology, while Doudna felt it was a joint project for which she was entitled equal recognition. Due to this conflict, Charpentier formed her own biotechnology company instead of joining Doudna (or Zhang).
Nevertheless, Doudna and Charpentier were jointly awarded several prizes for their work on CRISPR. Isaacson explains that these prizes affirmed two things: Doudna and Charpentier had made relatively equal contributions, and despite Zhang’s patent approval, they were the first to discover the technology. These affirmations were echoed by the greater scientific community when one of Zhang’s associates published an article lauding Zhang for his contributions to the discovery. Critics of the article argued that the article’s writer ignored Doudna’s contributions because he was sexist, which led to a Twitter firestorm.
Isaacson explains that since their application was still being processed when Zhang’s patent was awarded, Doudna and Charpentier were legally entitled to continue fighting for patent rights. They argued in court that they deserved the patent because they were the first to develop CRISPR gene editing technology and to say that it could be used in human cells. Zhang countered that he deserved the patent because Doudna and Charpentier’s original version of sgRNA didn’t work in humans and his innovation solved that problem. The US Patent Office determined Zhang should have the patent after all, but Doudna and Charpentier won other patent wars abroad, in nations like Mexico, China, New Zealand, and Japan.
How CRISPR Launched Doudna’s Career
Isaacson explains that despite her loss in the war for US patent rights, Doudna’s career has benefited tremendously from her contributions to CRISPR science and technology. He focuses on two primary benefits: First, Doudna entered the biotechnology industry. She started her own company (Caribou Biosciences), briefly worked for Editas Medicine with Zhang, and then returned to Caribou by joining its offshoot, Intellia. She then founded a research nonprofit (the Innovative Genomics Institute or IGI) and a company called Mammoth Biosciences, which both played an important role in the fight against Covid-19.
Second, Doudna and Charpentier were jointly awarded the Nobel Prize in Chemistry in 2020 for their research on CRISPR. Isaacson explains that their victory was historic for a few reasons. First, it usually takes decades for the Nobel Prize Committee to reward contributions to science. Second, there were only two recipients instead of the usual three—which means the committee decided that Zhang and other competitors were less deserving of credit for CRISPR gene editing technology. Finally, for the first time, all the recipients were women (and only five of nearly 200 previous recipients in history had been women). Doudna and Charpentier agreed that this was a monumental win for women and hoped it would inspire girls to pursue science.
Part 3: CRISPR Presents Moral Quandaries
Now that you know what CRISPR is and how it came to be, let’s discuss what its advent means for society. First, we’ll discuss the moral quandaries CRISPR gene editing presents. Then, we’ll explain how scientists and policymakers have addressed them so far.
Germline Editing
Isaacson says that most people think it’s morally OK to edit somatic cells—non-reproductive cells that affect only an existing person’s bodily composition. But people disagree about whether it’s OK to edit germline cells, which include eggs and sperm. When you edit germline cells, you genetically modify potential future offspring, and the changes you make could be inherited by their offspring as well. Isaacson describes a few opinions on each side of the debate.
Some people who are against germline editing argue that it’s wrong because it’s heretical or unnatural—either God or nature (via evolution) designed our genes the way they are for a reason, so humans shouldn’t interfere. Isaacson says this argument may not be logical: If nature or God endowed us with the ability to develop and use CRISPR, then using it can’t be unnatural or heretical. He also notes that genes aren’t distributed fairly—some people suffer more than others for no reason other than the luck of the genetic draw—and we may be morally obligated to even the playing field. However, Isaacson recognizes some existential risks of germline editing: We might develop hubris and become ungrateful for what nature or God gave us.
Some people who are in favor of germline editing argue that we have a moral duty to set our children up for success. Philosopher Julian Savulescu calls this stance “procreative beneficence.” Isaacson says that germline editing would accomplish this goal more efficiently than somatic editing. To illustrate, consider the blood disorder called sickle cell disease: Somatic edits can cure individuals, but germline edits could prevent their descendants from developing sickle cell disease in the first place. Theoretically, this would improve human life by leaps and bounds. But there’s also a downside to making germline edits—we might decrease genetic diversity, which is an evolutionary disadvantage.
Interventions vs. Enhancements
Isaacson says that scientists aren’t likely to abandon germline editing research, so society must determine under what conditions germline editing should occur. He describes a continuum of conditions that are under heavy debate by experts in the field. Many people believe germline editing is only OK when it serves as a medical intervention (with sickle cell, for example). They don’t believe it’s OK to unnecessarily enhance germline cells (like editing genes to make children conventionally attractive). They view genetic enhancements the way most people view the use of performance enhancement drugs in sports—they give people an unfair advantage and undermine the significance of talent, merit, and success.
But Isaacson explains that the boundary between interventions and enhancements is sometimes unclear—for example, acne is both a medical and cosmetic issue. This issue becomes even murkier when we consider that certain genes often have disadvantages and advantages. For example, inheritable mental illnesses are associated with higher creativity. If we edited out mental illnesses to reduce suffering, it might have a negative effect on the arts.
The Inequality Issue
Finally, Isaacson says that many people are concerned that the advent of human gene editing technology could exacerbate inequality. Gene editing therapies of all types—somatic and germline, interventions and enhancements—are likely to be extremely costly. Therefore, they’d only be available to wealthy people. In the case of germline edits, the edits would be inheritable, which means wealthy people’s descendants would be genetically advantaged. Over time, this could significantly widen the gap between rich and poor people—wealth and poverty would be inscribed in our genetic makeup, leading to clearly apparent differences in our features and abilities.
For this reason, writes Isaacson, some detractors argue that gene editing should be strictly regulated (if it’s allowed at all) so that it can only benefit society, not make it worse. On the other hand, some proponents of gene editing believe that free-market capitalism entitles us to make the best choices available to us given our individual means. For those on this side of the debate, individual freedoms outweigh any concern for the potential cumulative effects gene editing may have on society.
CRISPR-Related Policies
Isaacson explains that, when the field of bioengineering gained steam in the 1970s, scientists immediately recognized two threats. First, their research had serious consequences for society. Second, they might face government interference if they didn’t prepare for these consequences responsibly. Therefore, scientists gathered at conferences to create their own policies governing bioengineering research ethics.
After Doudna invented CRISPR gene editing technology, she had a nightmare that Adolf Hitler wanted to use it for nefarious purposes. Isaacson says that this dream, along with other fears about the potential consequences of her invention, led her to revive the tradition of science policymaking. She helped organize a 2015 conference where, after much debate, researchers concluded that germline gene editing research should be paused until scientists knew more about the risks it posed and could come up with safe, ethical research guidelines. However, germline gene editing research later resumed.
Part 4: CRISPR Applications
Regardless of the as-yet-unresolved moral quandaries that CRISPR presents, research involving CRISPR marches on full steam ahead. We’ll discuss CRISPR’s many applications—some that have already been realized and some that are yet to come.
Realized Applications of CRISPR
Isaacson describes many realized applications of CRISPR, but we’ll focus on three of the most momentous ones here.
First, in 2019, a Chinese scientist named He Jiankui created the first designer babies (modified humans created via germline gene editing). When the three babies were embryos, he edited a gene they had called CCR5, which makes you susceptible to HIV infection (but also protects you from West Nile Virus). The experiment was controversial because he blatantly disregarded the agreement science policymakers had made to pause germline editing. He faced international criticism and was convicted of criminal charges in China. His experiment led Doudna and other scientists to make a statement denouncing his actions and laying out guidelines for safer future experimentation with germline editing.
Second, CRISPR has been applied as a medical intervention in the form of somatic sickle cell gene therapies. This process involves taking stem cells from a sickle cell patient, editing the DNA in those cells to promote the production of healthy blood cells, and reinjecting the edited cells into the patient. Isaacson says that in 2020, the first patient to receive this treatment was cured of sickle cell disease. The treatment is expensive, and scientists believe germline sickle cell therapy could be more cost effective. However, extensive germline sickle cell therapy could be problematic: Most people with sickle cell disease live in parts of Africa where malaria is endemic, and the sickle cell gene protects you from malaria.
Finally, at the biotechnology organizations they founded, Doudna and Zhang spearheaded projects aimed at combating the Covid-19 pandemic. Isaacson says that bureaucratic obstacles prevented the US government from addressing the need for diagnostic tools quickly enough, so private scientists took the lead. Doudna and Zhang adapted diagnostic tools they’d created previously (to test for viruses like Zika and HPV) to diagnose Covid-19. They released simple, disposable, at-home tests for public use in April 2020 and freely shared their methods online so that anyone could use or enhance the technology. Doudna’s colleagues also invented a CRISPR-based vaccination method that may be adapted for use in future viral outbreaks.
Potential Applications of CRISPR
As we’ve mentioned, Isaacson explains that many of CRISPR’s realized applications will likely be enhanced and reused in the future (for example, CRISPR-derived tests and vaccines for Covid could be used to combat other viruses). He also notes that gene therapy trials for both viruses and diseases, including Huntington’s disease (a painful neurodegenerative condition), cancer, and congenital blindness, are already underway. Finally, Isaacson lists two other potential applications of CRISPR that could change the world: biohacking and bioweaponry.
Isaacson describes biohacking as a social movement aimed at democratizing biotechnology. One well-known biohacker, Josiah Zayner, sells CRISPR technology kits online, which enables anyone with the funds to buy one to do their own gene editing experiments—for example, one kit enables you to make bacteria bioluminescent. Zayner once publicly injected himself with a CRISPR solution to demonstrate his belief that everyone should have access to this technology. One benefit of expanding access is that as more people work with CRISPR, advancements will be made more quickly. But Isaacson implies that biohacking may also have serious risks.
Finally, Isaacson explains that many officials worry that CRISPR technology could be used to engineer bioweapons. For this reason, the US Department of Defense (DoD) sponsors research on anti-CRISPRs: naturally occurring systems that help viruses overcome CRISPR defenses in bacteria. Some scientists, including Doudna, are working on creating and implementing anti-CRISPR technology that could be used to fend off a CRISPR-derived bioweapon. Isaacson also notes that the DoD is currently pursuing research on the use of genetic enhancements to create supersoldiers.
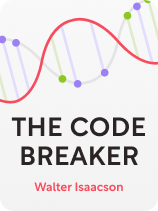
———End of Preview———
Like what you just read? Read the rest of the world's best book summary and analysis of Walter Isaacson's "The Code Breaker" at Shortform.
Here's what you'll find in our full The Code Breaker summary:
- The fascinating story of the groundbreaking CRISPR gene editing technology
- Why CRISPR presents scientists and citizens with serious moral quandaries
- How CRISPR has already changed life as we know it—and how it has the potential for even more