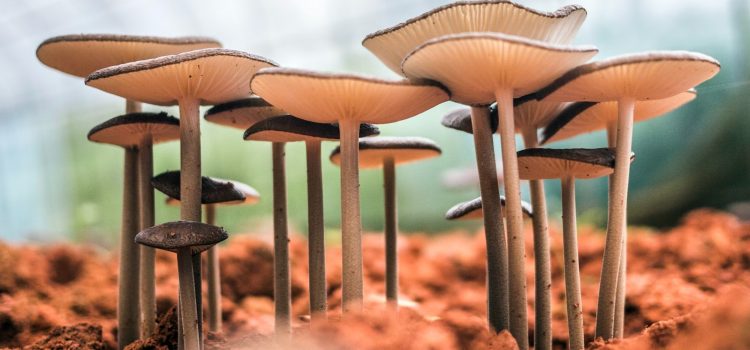
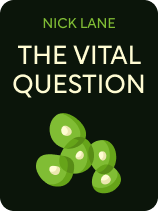
This article is an excerpt from the Shortform book guide to "The Vital Question" by Nick Lane. Shortform has the world's best summaries and analyses of books you should be reading.
Like this article? Sign up for a free trial here.
How did eukaryotes evolve? How can we even go about figuring it out?
Biochemist Nick Lane follows clues provided by introns to learn how eukaryotes evolved. He takes us on a journey through time, explaining how eukaryotic life might have experienced ever greater organization and complexity, eventually evolving into the plant and animal kingdoms we know today.
Keep reading to learn what Lane believes about the evolution of eukaryotes.
The Evolution of Eukaryotes
Because of the lack of intermediary steps on the evolutionary path to eukaryotes, it’s not easy to reconstruct the steps from that first symbiosis to the modern complex cell. But, just as Lane reverse-engineered the properties of the original cell, he’s able to do the same for eukaryotes, tracing our ancestors’ rapid evolution from a chimeric hybrid to a new branch of life. The clues about the evolution of eukaryotes that he draws upon are the introns—so-called “junk DNA”—that populate eukaryotic genomes but not that of bacteria and archaea. He shows that a sudden flood of such introns, stripped from bacteria in the eukaryotic host cell, populated the newly forming species and caused the evolution of the nucleus’s wall.
One thing that’s certain is that the nucleus evolved after endosymbiosis. Lane explains that we know this because a similar structure doesn’t exist in any species of archaea or bacteria. Also, because all eukaryotes have a nucleus, we know that it evolved relatively quickly—before eukaryotes had a chance to evolve into separate species. Evolution favors stability, and new adaptations only appear when absolutely needed for survival. Therefore, the merger of archaea and bacteria created an unstable internal situation that natural selection had to address quickly.
A Genetic Mosh Pit Lane mostly eschews a focus on genetics in favor of discussing life’s energy demands, but exploring the leap from archaea to eukaryotes requires understanding genetic competition and cooperation. In The Selfish Gene, evolutionary biologist Richard Dawkins states that living systems are merely the mechanisms by which genetic information perpetuates itself. He affirms that living systems have a natural preference for long-term stability and that they’ll strive to restore that stability as quickly as they can if their equilibrium is disrupted. Within a cell, few things could be more disruptive than a sudden influx of foreign DNA looking for a means to reproduce. However, genes don’t live in a dog-eat-dog world—for genes to survive, the host organism must survive. Therefore, while some genes compete directly with each other for survival, others have been seen to cooperate with each other for mutual benefit. None of this is conscious behavior—genes are just molecules, after all. Genetic cooperation is an artifact of evolution’s push toward self-replicating populations of genes with the best chance of survival. In the case of eukaryotes, that “best population” of genetic material takes shape in a cellular nucleus that deactivates counterproductive DNA. |
The instability of the original endosymbiotic merger is reflected in the chaotic state of eukaryotic DNA. Between useful strands of genetic information, the eukaryotic genome is full of dead lines of code—known as introns—that don’t produce any proteins at all. Lane traces the lineage of these introns to genetic parasites that inhabit bacterial DNA. These parasites aren’t living things themselves but are self-replicating bits of DNA that hitchhike inside bacteria and spread through lateral gene transfer. Bacteria and archaea have internal defenses such as enzymes that act as genetic pest control to stop these parasites and keep their numbers down.
(Shortform note: The genetic parasites Lane refers to are commonly known as transposons, and are found in modern eukaryotes such as plants and animals. These transposons can spread from one part of your genome to another by using your cells’ RNA messengers to insert themselves into different parts of your DNA. Eukaryotes such as ourselves aren’t susceptible to lateral gene transfer from other eukaryotic species, some animal species have absorbed genetic traits from symbiotic bacteria, and our genes can also acquire and spread transposons that travel as passengers inside viral infections. By actively rearranging DNA, transposons may be responsible for many of the mutations that drive evolution.)
Lane hypothesizes that something different happened during endosymbiosis. As the absorbed bacteria lost DNA to the archaeal host cell, it also released a flood of genetic parasites, and because they were parasites from bacterial DNA, the archaeal host cell had no defenses. These parasites, the precursors of introns, flooded the burgeoning eukaryotic genome and began to make use of excess ATP to generate a torrent of unusable proteins. Since the cell had ample energy, this wasn’t a problem until those proteins began to clutter the insides of the cell. Only then did evolution come up with a way to turn the introns off.
(Shortform note: Introns are different from exons, which are the sequences of DNA that actively code for the production of proteins. Not everyone agrees with Lane’s interpretation that introns are merely leftover bits of code from bacterial genetic parasites—some biologists argue that introns serve their own genetic functions in addition to the more commonly understood features of exons. These functions include regulating genetic transcription within the cell and organizing nested genes within DNA strands. It’s unlikely that introns served these functions when the original eukaryotic genome formed, but they were later repurposed through millions of years of evolutionary tinkering.)
The Nucleus Is Born
According to Lane’s proposal, our first eukaryotic ancestors evolved a way to shut down our introns by walling them off behind a membrane, the one that defines the outline of the nucleus. This membrane interrupts the process of expressing intron genes as useless protein strands while allowing the genes that should be active to carry out the tasks they’re assigned for. The nuclear membrane is able to do this because it’s based on bacterial DNA and can therefore defend against parasitic bacterial genetic code. While our ancestral bacteria became mitochondria, our ancestral archaea appropriated bacterial traits, such as the kind of protective membrane that could deactivate the introns blocking our single-celled forebears’ evolution.
(Shortform note: For the very reasons Lane describes, the integrity of the nuclear membrane is of vital importance to the cell. New research shows that the boundary of the nucleus is susceptible to being ruptured by viruses and congenital defects, which leaves the DNA inside exposed to potential chromosomal damage. Cancerous cells are particularly vulnerable to this kind of rupture, leading to additional genetic cell damage that may cause the cancer to mutate and spread further. Scientists have recently discovered that the protein vimentin may prevent this kind of rupture by fortifying the nucleus’s wall.)
With the formation of the nucleus and the birth of mitochondria, the evolutionary floodgates of eukaryotes were opened. The powerhouse of the cell and the flexibility of a large genome enabled the many evolutionary wonders of the natural world. Gone are the days when bacteria and archaea were the preeminent forms of life on the planet. All this, Lane says, took place because of a single instance of symbiosis that’s only happened once in the history of our world.
The Resilience of Eukaryotes The adaptive versatility of eukaryotes has been shown by how they repeatedly bounce back from mass extinction events as whole arrays of new species. While Lane argues that bacteria and archaea have never been threatened by the extinction-causing disasters that have periodically decimated more complex life, eukaryotes have had to claw their way back from the brink on multiple occasions. In The Sixth Extinction, Elizabeth Kolbert lists five prior global waves of extinction that have happened since eukaryotes first emerged on the macroscopic scale. In each of these, more than three-quarters of all eukaryotic life was extinguished, and yet every time, new species emerged with different, complex evolutionary solutions to the problem of surviving in the world they were born into—from trilobites to ancient sharks, dinosaurs, and us. Though many people such as Kolbert believe that we’re currently in the middle of another mass extinction, eukaryotic life will most likely survive and adapt to new forms long after we’re gone. |
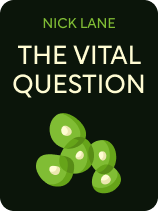
———End of Preview———
Like what you just read? Read the rest of the world's best book summary and analysis of Nick Lane's "The Vital Question" at Shortform.
Here's what you'll find in our full The Vital Question summary:
- Why genetics alone aren't enough to explain why cells function as they do
- A theory on where all complex life originated from
- An explanation of how cells work and are made