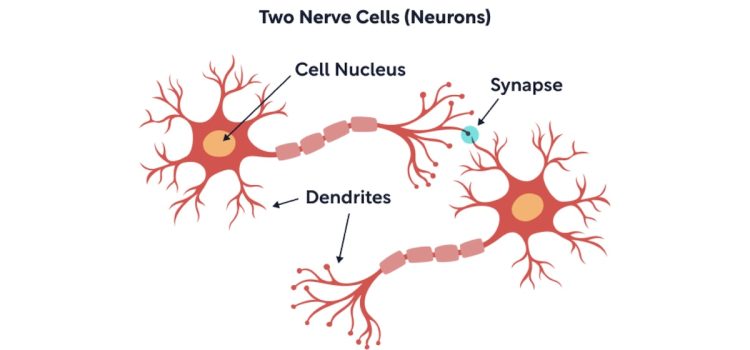
Do you understand how your brain processes information? What if your brain was actually made up of thousands of mini-brains working together?
In A Thousand Brains, neuroscientist Jeff Hawkins introduces the concept of cortical columns. These are small units in the brain that act like mini-brains, each processing information and making predictions. Hawkins explains how these columns work together to create our complex cognitive abilities.
Read more to learn how your brain might be more intricate than you ever imagined.
Cortical Columns
Hawkins proposes that the neocortex, the part of your brain responsible for higher cognitive functions, consists of around 150,000 cortical columns that each act as a mini-brain. Each column receives inputs, builds mental models, and makes predictions just as the brain does as a whole. The coordinated activity of these thousands of mini-brains working together produces the complex cognition and awareness that your brain is capable of.
(Shortform note: Hawkins attributes the discovery of cortical columns to Vernon Mountcastle in the 1950s, but as recently as 2005, neuroscience research had failed to establish a definitive purpose for these columns. The concept of a “column” itself wasn’t well-defined, with researchers using the term to refer to a variety of vertical cell clusters without a consensus on their significance. Furthermore, the existence and nature of cortical columns across different species is inconsistent, suggesting they may not be a fundamental unit of brain organization.)
Your cortical columns are highly interconnected, allowing them to integrate their outputs into a unified whole. Hawkins posits that each mini-brain communicates its findings to the rest, and through a process akin to voting, the cortical columns arrive at a consensus about what your senses detect and how you should react. Each column announces its perception and preferred reaction based on its individual input, and the brain’s overall response corresponds to the majority opinion of the columns. This produces what your conscious mind perceives as a unified experience despite the distributed nature of cortical processing.
From the high-level view of the brain, let’s zoom in on the neocortex. In a way, the neocortex looks like a crumpled-up towel, but if you were to flatten it out, you’d see that it’s a single sheet made of layers of nerve cells. Looking even closer at the flattened neocortex, we’ll find that it’s chiefly composed of vertical “columns” of cells that form circuits capable of making predictions and creating models of anything the brain encounters.
According to Hawkins, cortical columns are the fundamental information processing units of the neocortex. Each cortical column—a “mini-brain” in its own right—consists of a vertical arrangement of brain cells (neurons) spanning the horizontal layers of the neocortex. The human brain has approximately 150,000 cortical columns, and despite serving a variety of functions—such as interpreting speech or remembering directions—every cortical column operates according to the same basic principles. This uniformity suggests that the brain processes every type of information using a standard set of rules, making the study of cortical columns crucial for understanding brain function as a whole.
(Shortform note: Research on cortical columns, their structure, and their role in cognition blossomed in the 2010s. The neuroscience community is approaching a consensus that agrees with Hawkins’s characterization that these columns are the most basic building blocks of the cerebral cortex. However, there is still some debate about what actually constitutes a cortical column. Since the term’s introduction, its use hasn’t been consistent in scientific literature, sometimes referring to arrangements of nerve cells, and sometimes referring to other brain features. It’s also unclear how many cells they contain and how much they vary in design throughout the brain.)
Within a cortical column, neurons receive input through their dendrites—the finger-like extensions on the end of each nerve cell—and synapses—the points at which nerve cells connect. Neurons learn to “predict” certain inputs and react when inputs differ from their expectations. Hawkins explains that when multiple neurons in a cortical column receive the same unexpected input, they “fire” as one, sending signals to other columns throughout the brain. Since each neuron receives inputs from thousands of synapses, a single cortical column can process hundreds of inputs, make predictions, and generate responses at the same time.
(Shortform note: At the time of Hawkins’s book’s publication, neuroscience studies revealed more about the mechanism by which individual neurons learn to predict the inputs they receive. In essence, each neuron tries to maximize its impact on other neurons while minimizing its own energy consumption. In terms of biochemical efficiency, each neuron aims to minimize the difference between its actual activity and its predicted activity. When the actual amount of future activity differs from its prediction, a neuron updates its synaptic connections to improve its predictions for similar inputs. By continuously updating their input predictions, neurons become better at anticipating future activity patterns and reducing their overall energy use.)
Models in the Mind
However many inputs they learn, no single neuron can create what we’d consider a coherent thought. That’s why Hawkins asserts that clusters of neurons with thousands of connections are needed to fully interpret your senses and generate your mind and body’s reactions. Working together, these clusters of neurons create models of your environment, decide what to do and think based on those models, and learn more information about the world when your current models prove insufficient.
Hawkins suggests that, to make accurate predictions, cortical columns model objects and their positions in three-dimensional space using reference frames, which can be thought of like the grid lines on a map. His research shows that to create reference frames, neurons must be connected to both sensory input and motor output—it isn’t enough to see the world; your brain must be able to move through it. Different layers within a cortical column specialize in modeling objects and their positions separately—some neurons learn the shape of an object, while others determine where it exists. This modular setup lets the neocortex efficiently process and integrate information from your whole range of senses at once.
(Shortform note: Hawkins’s “reference frames” have been a staple of mathematics since René Descartes introduced the first x-, y-, and z-axis coordinate system in 1637. In the 1960s, computer pioneers began creating digital models of objects based on complex mathematical 3D reference frames, allowing users to interact with and manipulate imaginary objects on a computer screen just like your brain does when you think about an object and picture it in your own mind. Early computers didn’t “learn” 3D models—they were manually programmed by software engineers—but generative AI can now create 3D models based on simple text instructions, predicting how they interact and move, just as we do via models in the mind.)
These mental models form the foundation of all higher cognitive functions. Hawkins writes that as you interact with your environment, your neocortex continuously makes predictions based on your current mental models and compares its predictions to your sensory input. When predictions are accurate, they reinforce your existing models and strengthen their underlying neural connections. When predictions are wrong, your brain updates its models to better reflect reality. This constant cycle of prediction, feedback, and adjustment is the basis of learning, and according to the Thousand Brains theory, what’s happening on the brain’s macro level is actually taking place in each cortical column associated with a particular mental model.
(Shortform note: While Hawkins’s theory of learning via prediction and adjustment may seem straightforward, a certain amount of mental friction arises when our brains put it into practice. In Being Wrong, Kathryn Schulz explains that we’re programmed to assume our mental models are true, even in the face of insufficient or conflicting data. Confronting an error—or, an “incorrect prediction,” to use Hawkins’s term—elicits feelings of discomfort, remorse, and even shame. While our neurons may correct for inputs on the micro-level, when our large, complex mental models come under threat, our brains have a series of coping mechanisms akin to the stages of grief that they go through before correcting and adjusting their models of the world.)
Most of the predictions your brain makes are unconscious. Every time you move, such as by shifting your gaze or stepping into a room, your brain predicts what it expects to perceive. If nothing’s unexpected, you don’t notice anything—your cortical columns don’t light up and fire—but any surprises draw your attention and trigger your conscious brain to update its models. Hawkins says that since each cortical column contributes to hundreds of different mental models, they can switch between hundreds of different maps depending on your current sensory context. This flexibility allows your brain to navigate effectively through your ever-changing world.
(Shortform note: The process of learning by adapting mental models has been a mainstay of neuroscience even with older models of the brain. For instance, in Maps of Meaning, Jordan Peterson explains the same cycle in terms of left and right brain lateralization. He writes that as long as everything you encounter conforms to expectations, your brain’s “logical” left hemisphere is in charge. However, when the unexpected happens, the limbic system takes over, your senses become heightened, and your brain’s right hemisphere engages with its capacity for abstract, creative thought. In this interpretation, the right brain adjusts your mental models before passing them along to the left brain, which encodes them in language and logic.)
Can Cortical Columns Inspire AI Technology?
To overcome AI’s current limitations, Hawkins suggests that researchers could develop AI architectures that create reference frames and mental models in a way similar to how the cortical columns of the neocortex process information. These AI systems would learn by actively exploring and interacting with their environment, building and refining models based on sensory input and motor feedback. Reference frames, which are already used in robotics, could enable AI systems to build models of knowledge and relationships rather than the current AI approach which relies solely on statistical probabilities.
(Shortform note: Inspired by Hawkins’s theory about the brain, researchers at Carnegie Mellon University are developing Cortical Columns Computing Systems that aim to replicate the neocortex’s structure in digital hardware. By integrating attributes of cortical columns and their reference frames into electronic neural networks, they hope to create energy-efficient processing units capable of continual learning. In addition to mimicking brain-like cognition, this approach seeks to achieve brain-like efficiency in computing, which could potentially lead to significant improvements in how much power and energy AIs use compared to current machine learning systems with large environmental footprints.)